Introduction
Ondansetron (Zofran®) is a serotonin receptor (5-HTR3A) antagonist, which is used as an antiemetic. It was originally used was for nausea and vomiting after chemotherapy, irradiation, or surgery, but an extended off-label use has arisen, for hyperemesis and also nausea and vomiting in pregnancy (NVP) (Gideon Koren et al., 2012). Htr3 antagonists, granisetron (Kytril) and ondansetron, induce QT prolongation at high concentrations, leaving the patients with an elevated risk of cardiac dysrhythmias.1–4 However, the underlying mechanisms of QT prolongation by ondansetron during pregnancy are not well known.
During late pregnancy (LP), the maternal heart needs to adapt to significantly increased circulatory needs. Pregnancy-induced electrocardiogram disturbances are often observed, such as QT interval prolongation accompanied by down-regulation of Kv4.3, one of the key ion channels for repolarization.5 While all other serotonin receptor genes encode G-protein coupled serotonin receptors, the 5-HT receptor 3a gene (Htr3a) encodes subunits of the ligand-gated cation channels, forming a functional Htr3 channel.6–8 Recently, we reported that Htr3a knockout mice (Htr3a-/-) succumb to sudden death with fatal arrhythmia during pregnancy.1 In this study, we found that high serotonin levels initiated during pregnancy prevent severe QT prolongation during this period, by increasing the Kv current through Htr3a channels. The molecular mechanism underlying the sudden death and fatal arrhythmia during pregnancy in Htr3a-/- animals was associated with blocking the effect of serotonin on Kv current.
To understand the mechanism behind QT prolongation by ondansetron during pregnancy, we directly measured the effect of Htr3a antagonist and agonists on QT intervals and Kv currents in freshly isolated LV. Additionally, we evaluated the relationship between Kv4.3 and 5-HT3a using immunostaining. Finally, we evaluated the effect of ondansetron on the membrane trafficking of Kv4.3 in NP and LP mice.
Methods
This investigation conformed to the Guide for the Care and Use of Laboratory Animals published by the US National Institutes of Health (NIH Publication, 8 th Edition, 2011). Additionally, this study was approved by the Institutional Animal Care and Use Committee of Yonsei University, College of Medicine and Cardiovascular Research Institute, Seoul, Republic of Korea.
Optical mapping
Optical mapping was performed in 6 mice from each group. After performing a median sternotomy, the hearts were quickly excised. The hearts were stained with RH237 (Invitrogen, California, USA), and were excited with quasi-monochromatic light (520±30 nm) from two green LED lamps. A camera with a 610-nm long pass filter (MiCAM Ultima, BrainVision, Tokyo, Japan) was used to collect the emitted light at 1 ms/frame and 100 × 100 pixels with a spatial resolution of 0.5×0.5 mm2/pixel.8 To determine if the activation of Htr3 could shorten the action potential duration (APD), we measured the APD90 after infusion of serotonin (100 μmol/L), the Htr3 agonist, 1-(m-Chlorophenyl)-biguanide (m-CPBG, 200 nmol/L), and the Htr3 antagonist, ondansetron (1 μmol/L).
Single-cell electrophysiological recordings and data analysis
All physiological tests were performed during non-pregnant (NP) and late pregnant (LP; G16–18) stages. All procedures were performed after an anesthetic (isoflurane/O2) was administered via inhalation, and the heart was quickly removed after deep anesthesia was established. The heart was cannulated using a 24 G needle and then retrogradely perfused via the aorta on a Langendorff apparatus. During coronary perfusion, all perfusates were maintained at 37°C and equilibrated with 100% O2. Initially the heart was perfused with normal Tyrode solution for 2–3 min to clear the blood. This was followed by perfusion with a Ca2+ free solution for 3 min, after which, the heart was perfused with an enzyme solution containing 0.14 mg mL−1 collagenase (Yakult) in Ca2+ free solution for 12 min. After perfusion with the enzyme solution, the atria were separated from the ventricles, and chopped into small pieces. From these small pieces, single cells were dissociated in a high-K+ and low-Cl− solution using a blunt-tip glass pipette, and stored in the same solution at 4°C until use.
The recording protocols for Kv currents were also performed in ventricular myocytes, which were isolated from adult (8–10 weeks of age) C57BL6 mice as previously described.2 Briefly, we used pre-pulse to −40 mV for 25 ms to inactivate INa. Kv current was recorded in voltage-clamp mode with 400 ms pulses from a holding potential of −70 mV, with different test potentials increasing from −40 mV to +60 mV in 10 mV steps. The currents were normalized to the cell membrane capacitance (Cm) and averaged. The values were not corrected for the junction potential (pipette offset); this was compensated prior to giga-seal formation.
Cell membrane fractionation assay
Membrane isolation was conducted as explained earlier.3 All procedures were performed at 4°C. Ventricular myocytes were homogenized in a homogenization buffer (20 mmol/L Tris–HCl [pH 7.4], 1 mmol/L EDTA, and a protease inhibitor cocktail (2.5 μL/ mL, Sigma). Nuclei and debris were pelleted by centrifugation at 500 g for 10 min. The supernatant was further centrifuged at 25,000 g for 30 min. The pellet thus obtained was resuspended in homogenization buffer and centrifuged again at 25,000 g for 30 min. The final pellet was stored at −80°C. The protein concentration was determined by the Pierce BCA Protein Assay (Sigma-Aldrich).
Antibodies and immunoblot analysis
The primary antibodies used were anti-Kv4.3 (1:200, Santa Cruz Biotechnology), anti-Htr3a (SR-3A, 1:500, Santa Cruz Biotechnology), anti-pan cadherin (1:500, abcam), and anti-EEA1 (1:1000, BD Transduction Laboratories).
Cell lysates were separated by sodium dodecyl sulfate polyacrylamide gel electrophoresis (SDS-PAGE) and transferred onto nitrocellulose membranes (Bio-Rad, Richmond, CA, USA). After blocking with 5% non-fat dry milk in 1X tris-buffered saline (TBS) containing 1% Tween 20 (for 1h), the membrane was processed for immunoblot analysis with indicated primary antibodies. The primary antibodies were detected with horseradish peroxidase (HRP)-conjugated anti-rabbit or anti-goat immunoglobulin G (IgG) secondary antibodies. Detection was performed using an enhanced chemiluminescence detection system (ECL, Amersham Pharmacia Biotech, Piscataway, NJ, USA).
Confocal microscopy
Confocal microscopy was performed on isolated mouse ventricular myocytes as described previously.4 Briefly, ventricular myocytes were plated on laminin (10 μg/mL)-coated coverslips for 3 h at 4°C, fixed with 4% formaldehyde in phosphate-buffered saline (PBS) on ice, permeabilized in 2% donkey serum in PBS with 0.1% Triton X-100 for 30 min, and then incubated with the primary antibody against Kv4.3 (1:200, Santa Cruz Biotechnology) and Htr3a (SR-3A, 1:200, Santa Cruz Biotechnology), overnight at 4°C, followed by incubation with the Alexa Fluor 633-conjugated goat anti-mouse secondary antibody or Alexa Fluor 633-conjugated goat anti-rabbit secondary antibody and Alexa Fluor 488-conjugated donkey anti-goat secondary antibody at a 1:200 dilution for 1 h at room temperature. The immunostained cells were visualized using an LSM 700 confocal laser scanning microscope (Carl Zeiss). Cells were randomly selected and used for imaging and analysis, and immunostaining experiments were repeated at least five times.
Results
Serotonin shortens the QT interval and APD
To evaluate the effect of ondansetron on ventricular repolarization, we examined the effects of ondansetron on APD between NP and LP mice (Figure 1). When the animals were pretreated with an Htr3 antagonist, ondansetron (1 μmol/L), serotonin failed to shorten APD90. These results indicate that serotonin treatment shortens the APD and consequently the QT interval.
Figure 1.
Effect of ondansetron and serotonin on APD. A, Action potential tracings in NP (left panels) and WT-LP (right panel) mice treated with serotonin, m-CPBG, and ondansetron plus serotonin. (b) Comparison of the APD90 in WT-NP and WT-LP mice. Error bars indicate mean ± SD. The number of cells is indicated next to the symbols.
**P<0.01
APD90, action potential duration at 90%; LP, late pregnant; m-CPBG, m-chlorophenylbiguanide; NP, non-pregnant; OND, ondansetron; SD, standard deviation; WT, wild type

The effect of Htr3 antagonist on Kv current
We blocked serotonin receptors by applying ondansetron on freshly isolated LV cardiomyocytes from the NP and LP animals. Even at a high concentration, ondansetron (50 μM) did not decrease Kv current densities in NP mice. In contrast, ondansetron (50 μM) significantly decreased the Kv current densities in LP mice (44.3±1.9 vs. 35.8±2.7 pA/pF, p<0.01) (Figure 2).
Figure 2.
Effect of the Htr3 antagonist, ondansetron, on the Kv current in NP and LP mice LV myocytes. A, Kv current tracings from NP (upper panels) and LP (lower panels) mice LV myocytes, treated with control (CTR) buffer (left) and ondansetron (right). B, The effects of ondansetron on Kv current densities from NP and LP mice.
Kv, voltage-gated K+; LP, late pregnant; LV, left ventricular; NP, non-pregnant; OND, ondansetron

To measure the effect of ondansetron on the Kv current densities, LV myocytes were treated with various concentrations of ondansetron (0, 1, 5, 50 μM) for 10 min. As shown in Figure 3A, at higher concentrations (5, 50 μM), ondansetron significantly inhibited the Kv current densities.
Figure 3.
Effect of varying concentrations of ondansetron on the Kv current in LP mice LV myocytes. A, The effect of various concentrations of ondansetron on the Kv current. B, The analysis summarizes the effects of ondansetron on Kv current densities from LP mice.
CTR, control; Kv, voltage-gated K+; LP, late pregnant; LV, left ventricular; OND, ondansetron
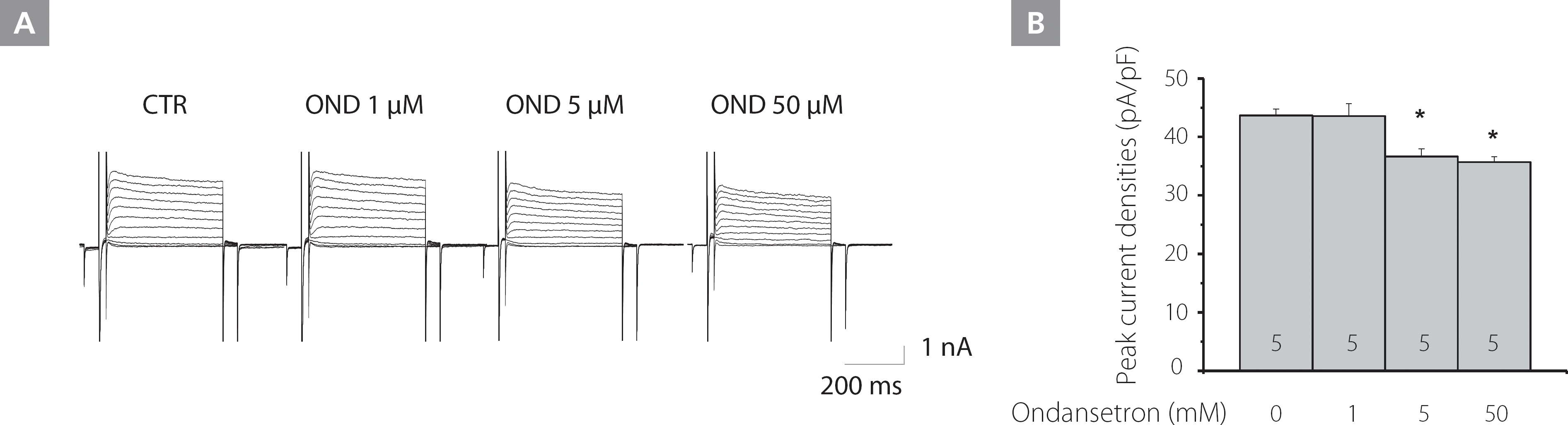
The effect of serotonin and an Htr3 agonist on Kv current
Kv channels control the height and duration of action potentials in the myocardium and are the primary determinants of action potential repolarization. To identify the molecular determinants of serotonin-induced APD shortening, we examined Kv channel properties; whole-cell patch clamp recordings were obtained from LV myocytes isolated from adult NP and LP mice. Figure 4A shows the waveforms of the Kv currents from NP (upper panels) and LP (lower panels) mice, respectively. Treatment with serotonin (100 μM) and m-CPBG (30 μM) for 10 min increased Kv current densities in NP, but not in LP mice. Co-application of ondansetron (50 μM) for 10 min blocked the increase in Kv current densities observed in NP mice caused by serotonin treatment.
Figure 4.
Effects of serotonin and Htr3 agonist on Kv currents in NP and LP mice myocytes. A, Kv current tracings from NP (upper panels) and LP (lower panels) LV myocytes treated with CTR buffer (a), serotonin (b), serotonin plus ondansetron (c), m-CPBG (c), and m-CPBG plus ondansetron (e). B, The analysis summarizes the effects of serotonin and serotonin plus ondansetron; m-CPBG and m-CPBG plus ondansetron on Kv current densities at +60 mV in NP and LP mice. The number of cells is indicated next to the symbols.
*P<0.01, ** P<0.001.
CTR, control; Kv, voltage-gated K+; LP, late pregnant; LV, left ventricular; m-CPBG, m-chlorophenylbiguanide; NP, non-pregnant; NS, not significant; OND, ondansetron

Figure 4B shows that serotonin increased the amplitude of peak Kv current densities (at +60 mV) in NP, but not in LP mice (55.0±2.5 vs. 72.7±4.9 pA/pF, p<0.001). Likewise, the Htr3a agonist, m-CPBG (30 μM), increased peak Kv current densities (at +60 mV) in NP mice, but not in LP mice (55.0±2.9 vs. 65.0±2.6 pA/pF, p<0.001). This data demonstrated that the Kv current was increased by serotonin and the agonist.
Serotonin activates Htr3a-mediated membrane trafficking
We evaluated the interaction between Kv4.3 and Htr3a in NP mice using confocal microscopy. Figure 5 revealed that Htr3a and Kv4.3 were co-localized on the surface of the membrane, T-tubules, and cytosol. With serotonin pre-treatment (100 μM) for 1 h, Kv4.3 and Htr3a were translocated to the surface membrane in NP mice. Co-application of ondansetron (50 μM) for 1 h erased the increase in membrane trafficking observed in NP mice caused by serotonin treatment.
Figure 5.
Enhanced Kv4.3 membrane trafficking in response to Htr3a-mediated serotonin stimulation in NP, but not in LP mice. A, Immunostaining of Kv4.3 and Htr3a in mice LV myocytes with Kv4.3 (green) and Htr3a (red); yellow indicates co-localization. The lower panels illustrate the optical density, along the white bar across the cells. Images are representative of at least 10 experiments. Scale bar, 20 μm. B, Comparison of the surface membrane/cytosol optical density ratio in NP mice. Bar graphs indicate the mean ± SD.
CTR, control; Kv, voltage-gated K+; LP, late pregnant; LV, left ventricular; NP, non-pregnant; NS, not significant; OND, ondansetron; SD, standard deviation

Because serotonin treatment caused the translocation of Kv4.3 and Htr3a to the membrane, we performed cell fractionation (see method). Serotonin and the agonist increased the abundance of Kv4.3 protein in the membrane fraction. On the other hand, co-application of serotonin with ondansetron (50 μM) for 1h erased this phenomenon in NP (Figure 6A [upper panel]), but not in LP mice (Figure 6A [lower panel]). This data supports the suggestion that the serotonin and agonist treatment caused the translocation of Kv4.3 and Htr3a to the membrane.
Figure 6.
Serotonin enhances membrane trafficking in NP, but not in LP mice. A, Plasma membrane preparations of LV myocytes with Kv4.3 showed greater Kv4.3 plasma membrane expression with serotonin and m-CPBG stimulation in NP (upper panels), but not in LP (lower panels) mice. This effect was abolished by co-incubation with ondansetron. Cytoplasm and the membrane components of LV myocytes were fractionated as described in “Experimental Procedures”, and the presence of each fraction was confirmed by immunoblotting with antibodies against specific marker proteins; pan-cadherin for plasma membrane; EEA1 for early cytoplasmic endosome. B, Blots are representative of 3 experiments with input and pharmacological interventions outlined above each panel. Bar graphs represent Kv4.3 expression levels. *P<0.01
EEA1, early endosome antigen 1; Kv, voltage-gated K+; LP, late pregnant; LV, left ventricular; m-CPBG, m-chlorophenylbiguanide; NP, non-pregnant; NS, not significant; OND, ondansetron

Discussion
Main findings
Ondansetron prolonged the QT interval with a decrease in Kv current densities and reduced the amplitude of peak current densities in a dose-dependent manner in the hearts obtained from pregnant mice, but not non-pregnant mice. During pregnancy, Htr3a-mediated Kv4.3 membrane trafficking was significantly impaired. This data suggests that ondansetron should be used cautiously during pregnancy, which is physiologically related with QT prolongation.
Ondansetron and ion channels
In this study, high concentrations of ondansetron decreased Kv current densities in pregnant animals. Voltage-dependent Na+ and K+ channels are important determinants of the human action potentials. Yuri et al. reported that the submicromolar affinity of ondansetron for the hERG K+ channel is likely responsible for the prolongation of cardiac repolarization by this drug (YURI A et al., 2000). However, its underlying mechanisms in other Kv channels have not been revealed. Moreover, even though this drug is frequently used as an antiemetic agent in pregnant women, the effect of ondansetron on cardiac repolarization during pregnancy has not been evaluated.
The maternal heart significantly adapts to the circulatory needs of pregnancy, but the effect of pregnancy on ventricular repolarization is poorly understood. Pregnancy is associated with electrocardiogram disturbances such as a longer QT interval. Eghbali et al.5,9 reported that Ito,f and IK,slow are down-regulated in pregnancy. In particular, cardiac Kv4.3 channel gene expression was down-regulated by 3- to 5-fold, and was accompanied by a reduction in the transient outward K+ currents, a longer action potential, and prolongation of the QT interval. Interestingly, in this study, the Htr3a antagonist, ondansetron, further decreased Kv current densities in pregnant mice. However, the effect of serotonin and the Htr3 agonist on cardiac repolarization was not observed in pregnant mice, whereas they caused shortened QT interval with increased Kv currents in non-pregnant mice. This finding suggests that although the effect of serotonin on cardiac repolarization is reduced, serotonin compensates for the QT prolongation during pregnancy by increasing Kv currents via Htr3.
Ondansetron and Kv 4.3 membrane trafficking
Unlike the other serotonin receptor genes, which encode G-protein coupled serotonin receptors, Htr3a encodes subunits of the ligand-gated cation channel, and is essential for the formation of functional Htr3 channels.6,8,106,8,10 The channel is predominantly selective for Na+ and K+, and its serotonin-activated opening depolarizes the cell membrane by permeating an inward Na+ current.11,12 The precise role of Htr3 as an ion channel expressed in the heart has not been fully evaluated. Our data demonstrates that serotonin increased Kv currents by increasing the membrane trafficking of Kv4.3 in non-pregnant mice. Therefore, it can be speculated that the effect of serotonin on cardiac repolarization was mainly mediated by an increase in Kv current via Htr3. However, this effect was not observed in NP mice pretreated with the Htr3 antagonist, ondansetron.
Study limitations
Our data shows that serotonin increases the Kv current densities via Htr3. Although we have demonstrated the relationship between the Htr3a and Kv channels using co-immunoprecipitation, it is still not clear whether the activated Htr3 increase influenced the Kv current densities directly or indirectly. Moreover, serotonin and Tph1 were increased during pregnancy. However, we could not exactly identify the source of the serotonin that affected the heart.
Conclusions
Ondansetron reduced Kv current densities, and reduced the Kv4.3 membrane trafficking in LP mouse ventricular cardiomyocytes. This data suggests that QT prolongation by ondansetron is mediated by the reduction of Kv current densities and Kv4.3 membrane trafficking. Moreover, ondansetron should be used cautiously during pregnancy, which is physiologically related with QT prolongation.