Atrial Fibrillation-Promoting Effect of Altered Left Atrial Calcium-Handling Proteins in Heart Failure
Article information
Abstract
Background and Objectives:
The incidence of atrial fibrillation (AF) is increased in heart failure (HF). Two major mechanisms of ventricular arrhythmia in HF are reentry mechanism due to tissue fibrosis and triggered activity due to an altered calcium-handling process. Unlike studies on ventricular arrhythmogenesis, only few studies investigated the relationship between changes in the calcium-handling process in atrial tissue and their relationship with atrial arrhythmogenesis in HF.
Materials and Methods:
HF was induced by myocardial infarction, and the rats were fed a normal sodium diet for 10 weeks and administered isoproterenol (30 μg/[kg∙h]) for the last 2 weeks of the experiment. Echocardiography and AF induction studies were conducted. In addition, mRNA and protein expressions of sarcoplasmic reticulum Ca2+-ATPase (SERCA), phospholamban (PLB), ryanodine receptor 2 (RyR2), and sodium calcium exchanger (NCX) were studied.
Results:
In the HF group, the ejection fractions were significantly decreased. AF duration (12.4±7.9 sec sham and 441.7±12.4 sec HF) and inducibility (8.0%±3.3% sham and 48.5%±10.6% HF) were significantly increased relative to the sham group. Atrial fibrosis tissue amounts were significantly increased in HF (0.20%±0.02% sham and 3.41%±0.45% HF). Atrial SERCA, PLB, RyR2 protein, and mRNA expressions were significantly decreased in HF, while the NCX protein expression was significantly increased in the HF group. The protein PLB/SERCA ratio was significantly increased by about 33% in the HF group.
Conclusion:
Changes in the protein and mRNA levels of calcium-handling proteins and increase in the degree of atrial fibrosis are important contributing factors of AF in severe HF.
Introduction
Atrial fibrillation (AF) is the most common cardiac arrhythmia. It is also a potent risk factor of ischemic stroke [1]. The clinical risk factors of AF include advanced age, diabetes, hypertension, congestive heart failure (CHF), valvular heart disease, and myocardial infarction (MI) [2-4].
The incidence of AF is increased in CHF, and asymptomatic left ventricular (LV) dysfunction is associated with an increased risk of AF [5,6]. There are two mechanisms of arrhythmias in HF, one is the reentry mechanism, which is associated with increased tissue fibrosis [7], and the other is triggered activity, which is associated with HF-induced ionic remodeling, increased sodium-calcium exchanger, and reduced inward potassium current [8].
Abnormal intracellular Ca2+ cycling plays an important role in cardiac dysfunction and ventricular arrhythmogenesis in the setting of heart failure (HF), myocardial infarction (MI), and ischemia reperfusion (I/R) injury [9]. Many studies have been conducted about the changes in the levels of ventricular calciumhandling proteins in HF and their relationship with ventricular arrhythmia. However, changes in the expressions of genes encoding calcium-handling proteins in the context of atrial arrhythmia and HF have not been characterized. Although the mechanisms of atrial arrhythmogenesis have been traditionally evaluated by using electrophysiological methods, especially the patch-clamp technique, translational and transcriptional changes in the expression levels of genes encoding calcium-handling proteins in atrial tissue have not been studied yet.
We, therefore, investigated the relationship between changes in the levels of calcium-handling proteins and subsequent AF development in a rat model of severe HF.
Materials and Methods
Animals and Preparation
All animal-handling procedures were approved by the Animal Research Ethics Committee of the Kosin University School of Medicine, which follows the guidelines of the Korean Council on Animal Care. Male Sprague-Dawley (SD) rats (Daehan Biolink Inc., South Korea) aged 7 to 8 weeks, weighing 250 to 300 g, were fed a normal sodium diet and administered tap water. Two days after the MI, the rats were randomized into two groups, namely the HF and sham groups. In the HF group, 8 weeks after MI induction, to develop a more severe HF, isoproterenol (30 μg/[kg∙h]) was continually infused through an Alzet osmotic pump (Durect, Canada) for 2 weeks. Sham-operated rats served as controls.
Creating the MI Rat Models
MI was induced in the SD rats after ligation of the left anterior descending coronary artery under anesthesia induced by intraperItoneal injection of ketamine (50 mg/kg) with xylazine (10 mg/kg). The rats were connected to a respirator (Harvard Model 683 Rodent Ventilator) with a frequency of 60 ventilations per minute and a volume of 1 mL/100 g). The chest was opened using mid-sternotomy, and the left anterior descending coronary artery was ligated approximately 2 to 3 mm from its origin with a 5.0-polypropylene suture (Ethicon, Belgium). The control rats were submitted to a sham operation by using a similar procedure without coronary ligation. The perioperative mortality was around 40% in the rats submitted to the coronary artery ligation.
Echocardiography
At the end of the study, the rats were lightly anesthetized with an intraperItoneal injection of ketamine (25 mg/kg) with xylazine (5 mg/kg). Transthoracic echocardiography was performed by using Acuson Sequoia C512, a 12-MHz phased-array probe (Mountain View, CA, USA). Wall thickness and LV diameters during diastole and systole were measured from M-mode recordings according to the leading-edge method. Two-dimensional short-axis images at the mid-papillary muscle level were obtained for the measurement of LV end-diastolic dimension, LV end-systolic dimension, fractional shortening, and wall thickness. Ejection fraction (EF) was calculated as follows: EF = (D2 - S2)/D2 × 100, where S was the systolic dimension and D was the diastolic dimension (expressed in centimeters). LA and aorta diameters were measured from the M-mode recordings in a modified parasternal long-axis view.
Electrophysiological Study
For the open-chest study, in the case of HF, we waited for 3 or 4 days after removal of the osmotic pumps. The rats were anesthetized with ketamine (90 mg/kg) and xylazine (13 mg/kg), and ventilated mechanically. A bipolar lead was attached to the right atrial appendage, and AF was induced by right atrial burst pacing (4 times the pacing threshold for 30 sec at 600 bpm). AF was induced ten times if the AF duration was ≤20 min and five times if the AF lasted between 20 and 30 min. AF that lasted more than 30 min was considered to be persistent and recorded as 30 min in duration. The mean duration of AF in each rat was determined.
Quantification of Fibrosis
After the completion of the electrophysiological study, the heart was rapidly removed. The hearts were immersed in a 10% buffered formalin solution and then embedded in paraffin. Masson trichrome staining was used to characterize the collagen fibers. Fibrosis of the left atria was quantified with the acquisition software Image-Pro Plus version 5.1 (Media Cybernetics, Georgia, USA).
Western Blot
About 25 mg of rat left atrial tissue was homogenized in 400 μL of a modified tonic sucrose solution (0.3 mol/L sucrose, 10 mmol/L imidazole, 10 mmol/L sodium metabisulfite, 1 mmol/L DTT, and 0.3 mmol/L PMSF) and centrifuged at 1300 × g at 4°C for 15 min. Protein concentration was determined by using the Bradford method. Aliquots containing 25 μg of protein for the SERCA and phospholamban (PLB) analyses, and 40 μg of protein for the Na/Ca exchanger (NCX) and RyR2 analyses were loaded on the sodium dodecyl sulfate-polyacrylamide gel and separated by electrophoresis (10%, 13%, and 4% acrylamide separating gel for 90 min or 4 h [RyR2], respectively). The separated proteins were electrophoretically transferred onto a polyvinylidene fluoride membrane (Pierce Rockford, IL, USA) at 25 V for 90 min or at 10 V for 12 h (RyR2) in a buffer containing 25 mmol/L Tris base, 192 mmol/L glycine, and 20% methanol or no methanol (RyR2) by using a Trans-Blot semi-dry transfer system (Bio-Rad, Hercules, CA, USA). The membranes were incubated at 4°C overnight with mouse-anti rat-PLB (Affinity BioReagents, Golden, USA), RyR2 (Affinity BioReagents), NCX (Affinity BioReagents) monoclonal antibodies, and rabbit-anti-rat-SERCA (Santa Cruz, California, USA) polyclonal antibodies at a 1:1000 dilution in TBST (0.2 mol/L Tris, 1.37 mol/L NaCl, 0.1% Tween 20; adjusted pH 7.6) containing 5% skim milk. After incubation with a secondary antibody at a 1:1500 dilution in a TBST containing 5% skim milk, the blot was developed by using a SuperSignal West Pico Chemiluminescent Substrate (Pierce, Rockford, IL, USA) and exposed to an X-ray film. DensItometric analysis of the western blot was performed. All of the results were normalized to glyceraldehyde-3-phosphate dehydrogenase (GAPDH) protein level data obtained from the same samples at the same time.
Real-Time Quantitative Reverse Transcriptase Polymerase Chain Reaction
Total RNA was isolated from 10-20 mg of rat left atrium tissues with Trizol reagent (Invitrogen, USA), followed by chloroform extraction and isopropanol precipitation. Genomic DNA was eliminated by incubation in rDNase I (2 U/μL, 37°C, Ambion, USA) for 30 min. RNA was quantified by spectrophotometric absorbency (260 nm). Purity was confirmed by the A260/A280 ratio.
Gene-specific primers for real-time reverse transcriptase polymerase chain reaction were designed based on published cDNA sequences for rat SERCA, PLB, NCX, and RyR2, respectively.10 First-strand cDNA (synthesized by reverse transcription with rat atrial mRNA samples) was used as a template for subsequent real time PCR. Two-step real time PCR was conducted with the LightCycler® Real-Time RT-PCR system (Roche Applied Science).
The primers used for the detection of SERCA, PLB, NCX, RyR2, and beta-actin were as follows: forward primer 5'-AAG-CAG-TTC-ATC-CGC-TAC-CT-3' and reverse primer 5'-AGA-CCA-TCC-GTC-ACC-AGA-TT-3' for SERCA; forward primer 5'-TAC-CTT-ACT-CGC-TCG-GCT-ATC-3' and reverse primer 5'-CAG-AAG-CAC-AAT-GAT-GCA-G-3' for PLB; forward primer 5'-TGA-CAA-TTT-CTT-AGA-TGG-CGC-T-3' and reverse primer 5'-TGC-TGC-TGA-CTT-AGG-ACT-TGA-T-3' for NCX; forward primer 5'-ACT-GCT-AAA-GTG-ACC-AAC-AG-3' and reverse primer 5'-TTG-CAT-CGC-TGA-AAT-CTA-GT-3' for RyR2; and forward primer 5'-GTA-CCA-CTG-GCA-TTG-TGA-T-3' and reverse primer 5'-CTG-TGG-TGG-TGA-AGC-TGT-A-3' for betaactin. Real-time PCR was run in the presence of a double-stranded DNA-binding dye (SYBR). Beta-actin was used as an internal standard, and all results were normalized to beta-actin data obtained from the same samples at the same time. Total RNA was run in duplicate for each experiment.
Statistical Analysis
The statistical analysis for each parameter studied was performed with Kruskal-Wallis testing. If the Kruskal-Wallis tests were significant, group-to-group comparisons were performed by using the Mann-Whitney test. p<0.05 was considered statistically significant. All results are expressed as the mean ± standard error of the mean (SE) unless indicated. For the statistical analysis, the SPSS software (version 12.0K) was used.
Results
Echocardiographic Indexes
Echocardiographic parameter changes in each group are presented in Figure 1 and Table 1. The LV ejection fraction significantly decreased from 79.7%±2.1% (sham, n=15) to 39.2%±3.3% (HF, n=15; p<0.001, Figure 1, Table 1). LV end diastolic and left atrium dimensions were significantly increased in the HF group.
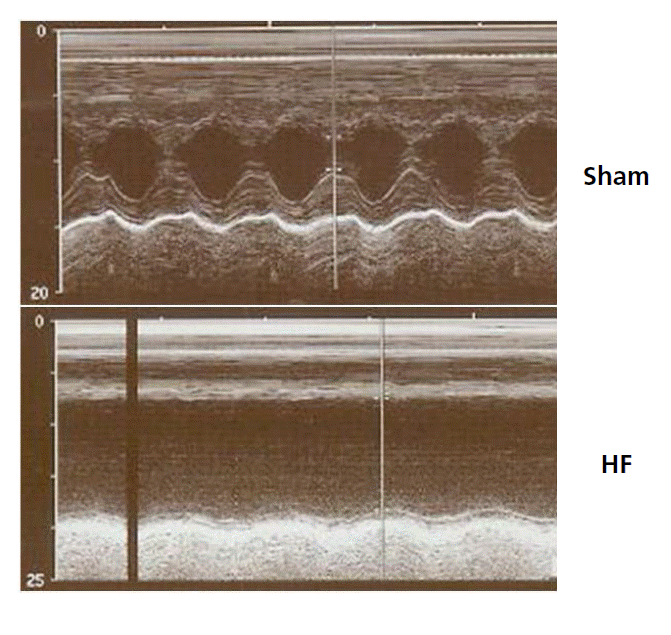
Echocardiograms shows left ventricular anteroseptal wall akinesia, posterior wall hypokinesia, and dilated ventricular dimensions in heart failure rats.
HF, heart failure.
Heart Weight-To-Body Weight Ratio
MI and isoproterenol infusion increased heart weight significantly in the HF group compared with the sham (sham, 3.37±0.13 mg/g, n=10; HF, 5.60±0.22 mg/g, n=10; p<0.001, Figure 2).
AF Induction Study
AF inducibility was significantly increased in the HF group compared with the sham group (sham, 8.0%±3.3%, n=15; HF, 48.5%±10.6%, n=13; p<0.001). In addition, AF duration significantly increased in the HF group (441.7±12.4 sec, n=13, compared with the sham 12.4±7.9 sec, n=15; p=0.015, Figure 3, Figure 4).

The electrocardiogram shows the successful induction of atrial fibrillation following burst pacing in the heart failure group. On the contrary there is no induction of atrial fibrillation in the sham group. AF, atrial fibrillation; HF, heart failure.
Left Atrial Fibrosis Amount Analysis
Interstitial fibrosis in the left atrium (sham, 0.20%±0.02%, n=5; HF, 3.41%±0.45%, n=5; p=0.008) was significantly increased in the HF group (Figure 5).
Expressions of Calcium-Handling Proteins
The left atrial tissue protein levels of SERCA, PLB, and RyR2 were significantly decreased in the HF group (45%±7%, 60%±3%, and 58%±9%, respectively) compared with the sham group (100%±6%, 100%±5%, and 100%±7%, respectively; p<0.001 for SERCA and PLB, and p=0.016 for RyR2). However, the NCX protein expression level was significantly increased in the HF group (183%±16%) compared with the sham group (100%±5%; p<0.001). The PLB/SERCA ratio was increased by about 35% in the HF (133%±7%) compared with the sham group (100%±5%; p<0.001, Figure 6.). All results were normalized to GAPDH data concomitantly obtained from the same samples.
Changes in the Transcriptional Levels of CalciumHandling Genes
The left atrial tissue mRNA levels of SERCA, PLB, and RyR2 were significantly decreased in the HF group as compared with the sham group (p<0.001 for SERCA and RyR2, p=0.023 for PLB). However, NCX was increased in the HF group, but the increase did not achieve statistical significance (p=0.105). The PLB/SERCA ratio was significantly increased in the HF group (9.39±4.76; p<0.001, Figure 7).

Relative mRNA expression of calcium handling genes in the left atrium. Data represent mean ± SE in each group, *p<0.05, **p<0.001 sham vs. HF HF, heart failure; mRNA, messenger ribonucleic acid; NCX, sodium calcium exchanger; PLB, phospholamban; RyR2, ryanodin receptor 2; SERCA, sarcoplasmic reticulum Ca2+-ATPase.
Discussion
Main Findings
In the present study, we found altered calcium-handling protein levels, namely decreased SERCA2a, PLB and RyR2 protein, and mRNA expression levels, and an increased PLB/SERCA ratio in HF rat atrial tissue. The NCX protein expression was increased. We also found significantly increased fibrosis in HF. These findings were associated with increased AF inducibility and AF duration in the HF group.
Altered Calcium-Handling Protein Expressions and Arrhythmogenesis
In HF ventricular cardiomyocytes, action potential (AP) is usually prolonged and SR Ca2+ release is usually reduced [11]. Transient outward current (Ito) and inward rectifying K+ current (IK1) are decreased, and an increase in late sodium current prolongs the action potential in HF ventricular myocytes [12,13]. Reduced Ca2+ uptake into the SR, increased Ca2+ removal via the NCX, and enhanced diastolic Ca2+ leak from the SR occurs in HF [14]. This alteration in the calcium-handling process is a major mechanism for delayed after depolarization (DAD) and early after depolarization (EAD). EAD is associated with adrenergic stimulation and related to AP prolongation and increase in Ca2+ window currents. Increased Ca2+ leak during diastole downregulates IK1, and increased NCX levels facilitates the initiation of DADs.
Previous studies about calcium-handling proteins have shown that CHF is accompanied by decreased SERCA expression level, increased PLB/SERCA ratio, and SR functional deficiency in ventricular tissues [9,15-19]. These changes may cause the depressed SR Ca2+ load, which is believed to be a major cause for the contractile defect and arrhythmogenesis [20]. PLB is an integral protein of the SR of mammalian myocardium and regulates SERCA activity. The unphosphorylated state of PLB inhibits SERCA activity [21].
In this study, we observed downregulated SERCA2a and PLB and upregulated PLB/SERCA2a ratio in CHF atrial tissue. This defective Ca2+ uptake into SR causes decreased systolic calcium release from SR, increased cytosolic free calcium level, and subsequent depressed contractile function, and increases vulnerability to arrhythmia.
Calcium is released from the SR via the ryanodine receptor (RyR2). It was reported that RyR2 mRNA levels were reduced in HF in both human and animal models [22,23]. This reduced ryanodine receptor level is associated with SR calcium leakage and contractile dysfunction [24].
In this study, we observed downregulated RyR2 protein and mRNA, and upregulated NCX protein levels in HF. These are associated with SR calcium leakage in atrial cardiomyocytes, atrial contractile dysfunction, and arrhythmogenesis.
The removal of calcium from the cytosol by sarcolemmal NCX, which has high capacity and low affinity, is the major calcium extrusion mechanism of the cardiac myocyte [25]. NCX, known as an important carrier of post-repolarization transient inward currents, causes DADs, which can induce triggered activity. Evidence supports the role of DADs in atrial tachyarrhythmias in canine CHF models [26]. Most studies consistently report that NCX expression is increased in CHF ventricular cardiomyocytes. In this study, we also found increased NCX protein expression in HF atrial tissue. We posit that increased NCX related to DADs plays an important role in developing atrial arrhythmia in HF atrial tissue.
HF and β-Adrenergic Stimulation-Induced Atrial Fibrosis and Its Relationship with AF Vulnerability
In this study, we used isoproterenol to generate severe HF. Because advanced HF is associated with increased sympathetic activity, we used an advanced HF model with isoproterenol juxtaposed on a post-MI HF model. Isoproterenol-infused postMI HF was associated with severe left atrial interstitial fibrosis. Subsequently, an increased amount of atrial interstitial fibrosis can be a substrate for AF development and maintenance by the reentry mechanism.
Study Strengths and Potential Limitations
We did not measure calcium transient, calcium spark, and L-type calcium current because of the poor condition after isolation of single atrial cardiomyocytes in HF. Furthermore, we could not measure the phosphorylation states of PLB, RyR2, and calmodulin-dependent protein kinase II because of the long study period and small amount of tissue obtained from the rat atrium.
However, our study provides, to our knowledge, the first report of the evaluating transcriptional and translational changes of atrial calcium-handling proteins in atrial arrhythmia in CHF. These results are important to understand the mechanisms and potential treatment of AF.
Acknowledgements
Part of this study was supported by the 2005 research grant of Kosin University College of Medicine, Busan, South Korea.